Stem cell therapies, making a comeback? - Reeve Foundation
In recent weeks, there’s been a renewed buzz around stem cell therapies for SCI. I wanted to take my first research blog post to break down some of the stem cell SCI research, and do a deeper dive into one paper, 2019 (Shiga et al) out of Wendy Campana’s lab at UCSD.
For starters, some definitions. The first stem cells explored for neurological research were embryonic neural stem cells (NSCs), those derived from embryonic tissue, for their capacity to develop into multiple neuron types and connect to targets appropriately (remember, adult neurons do not divide and grow like other cell types). These NSCs showed promise in preclinical rodent models of SCI, but stalled in translation for a multitude of reasons. An alternative source of stem cells are those derived from adult skin, dental pulp, fat, or blood cells, reprogrammed with chemical and environmental steps to become neural progenitor cells. Due to their reprogramming, these are called induced pluripotent stem cells, or iPSCs. iPSCs have the ability to be created from a patient’s own cells, thus avoiding the massive immune response generated when foreign cells are introduced. iPSCs are somewhat of a blank slate, they can become neurons, or heart cells, or skin cells, etc, so researchers may want to chart them on a neural course. Researchers here use the term human induced neural progenitor cells (hiNPCs), used to describe iPSCs guided to a neural identity.
One of the things you’ve likely already gathered, is that all of these steps to induce and reprogram cells essentially require researchers to follow a very specific recipe. That recipe often differs between research groups, and may underlie both the success of transplantation and extent of functional improvement. Sometimes iPSCs don’t even need to become neurons to have meaningful benefit (e.g. oligodendrocytes, glia), they might help coat neurons to improve their function or release substances that improve the spinal cord’s ability to repair itself. Yet if we want them to become new functional neurons in the spinal cord, when we implant iPSCs into a subject (human or animal), we want them to survive, grow, and connect in a meaningful manner.
Here’s where I think the Shiga et al paper is most interesting. They took a severe crush injury in rats, and instead of implanting any old iPSC, they took human iPSCs reprogrammed for a neural identity (hiNPCs), and pretreated them with a common stroke drug, tissue plasminogen activator tPA. tPA has growth factor like properties, can interact with neurotransmitter receptors, and can impact immune reactions, as well as enzymatic activity. In order to keep the pro-growth impact of tPA and avoid any enzymatic activity, researchers incubated hiNPCs with an enzymatically inactive form of tPA, before injecting them into SCI rats 1 week after injury. They had 3 groups: an SCI only group, a stem cell (hiNPC) group, and a stem cell pre-treated with the stroke drug (tPA-hiNPC) group, and measured both pain and motor behavior in the months after implantation. While they saw no difference in pain sensitivity, they saw a remarkable improvement in motor behavior (graph below).
The Basso, Beattie and Bresnahan (BBB) locomotor rating scale was used to assess motor function. An improvement from approximately 5 to 15 is the ability to both weight support and walk around their cage, a meaningful increase in score. Researchers also found that many of these stem cells survived, held a mature neuron identity, and extended axons up to 4 segments below the level of injury 2-4 months post injection.
It’s still unknown whether this sort of strategy will have success in chronic SCI, or whether pre-treatment of subject-derived hiNPCs is a viable method for humans (or if there may be some sort of small molecule that can mimic the effects of pre-treatment). Yet, I wanted to highlight this study as one of the stem cell animal studies that seeks to answer the appropriate questions: (1) what is the best type and condition of stem cell to implant, (2) can we do anything to improve the chance of success for the stem cells to appropriately implant, survive, and grow; (3) does treatment result in meaningful, functional improvement, (4) what do the stem cells become (non-neural cells, spinal cord neurons, motor neurons) as time progresses post implantation, and (5) are there any obvious detrimental side effects after implantation? To fully answer (5), researchers might need to assess many other functions, including chronic pain and autonomic function, over a longer time window, but this is a good start. I’m looking forward to seeing where this group goes next.
Join Our Movement
What started as an idea has become a national movement. With your support, we can influence policy and inspire lasting change.
Become an Advocate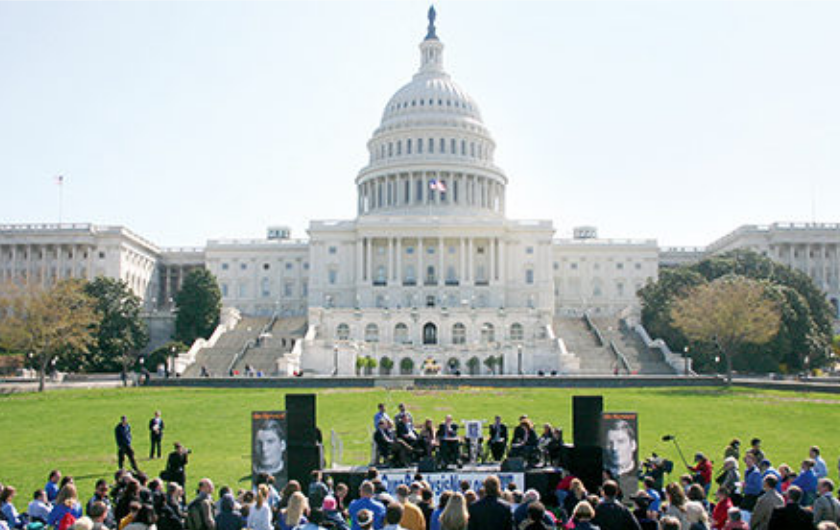